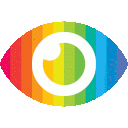
1. Researchers have developed a plasmo-photonic platform using CMOS processes that integrates single-mode aluminum (Al) plasmonic stripes with a low-loss Si3N4-based photonic waveguide platform.
2. The Al-based plasmonic waveguides demonstrated the highest propagation lengths among all single-mode Al-based plasmonic waveguide structures presented so far at 1550 nm, making them suitable for on-chip data transmission and biochemical sensing applications.
3. The proposed co-integration scheme exploits the optical functions provided by the low-loss Si3N4 platform, facilitating fiber-to-chip coupling through grating couplers and allowing on-chip excitation of surface plasmon polariton modes through a butt-coupled Al-to-Si3N4 interface.
The article discusses the development of plasmonic waveguide circuits using CMOS compatible metals, specifically aluminum (Al), co-integrated with a low-loss photonic platform. The authors highlight the challenges associated with other CMOS compatible metals like copper and titanium nitride, which have either stringent manufacturing requirements or lower plasmonic propagation lengths compared to noble metals. The article presents experimental measurements of plasmonic propagation losses in air and water, as well as signal integrity credentials for Al-based plasmonic waveguides transmitting modulated optical data at 25 Gb/s.
Overall, the article provides a detailed account of the research conducted by the authors and their findings. However, there are some potential biases and limitations to consider. Firstly, the authors only focus on Al as the plasmonic material of choice without exploring other CMOS compatible metals in depth. While they acknowledge that promising results have been reported for copper and titanium nitride, they do not provide a comprehensive comparison of these materials' properties and suitability for different applications.
Secondly, while the authors claim that their co-integrated plasmo-photonic platform is entirely manufactured using CMOS processes, they do not provide details on any potential risks associated with these processes or their impact on the environment. Additionally, they do not explore any potential limitations or drawbacks of using CMOS processes for plasmonics.
Thirdly, while the authors present experimental measurements of plasmonic propagation losses in air and water, they do not provide any evidence for their claims regarding Al's durability to liquid claddings or its suitability for biosensing applications. They also do not explore any potential limitations or challenges associated with using Al-based plasmonic waveguides in biochemical sensing configurations.
Finally, while the authors present signal integrity credentials for Al-based plasmonic waveguides transmitting modulated optical data at 25 Gb/s, they do not explore any potential counterarguments or limitations to their findings. They also do not provide any evidence for the scalability of their technology or its potential impact on the field of plasmonics.
In conclusion, while the article provides valuable insights into the development of plasmonic waveguide circuits using CMOS compatible metals, there are some potential biases and limitations to consider. The authors' focus on Al as the plasmonic material of choice and their limited exploration of other CMOS compatible metals, as well as their lack of discussion on potential risks associated with CMOS processes and their impact on the environment, may limit the applicability and scalability of their technology. Additionally, while they present experimental measurements of plasmonic propagation losses and signal integrity credentials for Al-based plasmonic waveguides, they do not explore any potential counterarguments or limitations to their findings.